
Photosynthesis
Photosynthesis:
Plants are examples of “Autotrophs,” or, organisms that can support themselves and don’t need to eat other things (we are “heterotrophs;” we do eat other things). In particular, plants are “photoautotrophs,” supporting themselves with light.
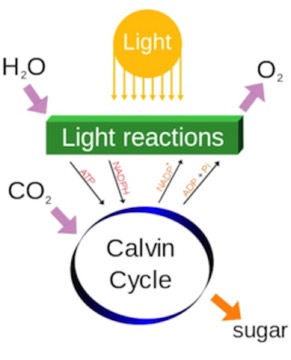
The ATP and reduced nucleotide cofactor (NADPH) are used in the synthesis part (Calvin Cycle) to pull CO2 out of the air and synthesize carbohydrates. In the process, the oxidized NADP+ and ADP are produced…and fed back into the light reactions.
Light Reactions
The general idea is that photons of light hit electrons, exciting them to a higher energy level. Instead of “falling” back down and emitting light, the high energy electrons are passed to other molecules, reducing them, and the electrons are passed down an electron transport chain of proteins or smaller molecules that are reduced, then oxidized by the next player in the chain. In this way, just like in respiration, protons are pumped across the membrane and those drive the ATP synthase. The first recipient in the chain is called “Plastoquinone” and it looks like this:
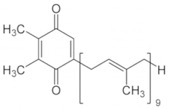
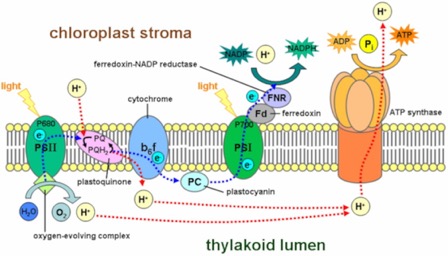
Some key points:
- In the Z scheme, PSII is excited “first,” (there are many reaction centers all being hit more or less simultaneously. It’s just that to see the “scheme,” we have to imagine things happening in sequence).
- Electrons leave PSII. They are replaced by electrons taken from oxygen in water (a rare case of oxygen being oxidized). This generates O2, which is good for us.
- At some point PSI also gets hit by a photon of the right energy. That electron can be passed directly to an iron-containing protein called “Ferrodoxin” and eventually used to reduce NADP+. The electrons are replaced by electrons coming down the from the PSII system.
- The Z scheme is also called “linear flow” of electrons because they appear to start at one place (PSII) and pass down the chain, eventually leaving the system. You could call it an “open” pathway, since electrons will leave the system at the end, transferred to NADP+ to make the reduced NADPH, and therefore need to be replaced by electrons from oxygen, obtained in PSII.
- Photosystem 1 (PSI) can work on its own without PSII. In that case the electrons excited from PSI get passed down the chain to Cytochrome b6f and passed back into a PSII reaction center. When this happens: Oxygen is NOT released and NADPH is not made. In some organisms, the electrons can be taken from some other source and NADPH can be made, but, oxygen is not produced. (So, it is not necessarily true that you need both systems to perform the "synthesis" part.
- From an evolutionary point of view, PSI may be older. If that’s the case, photosynthesis was going on for a long time without oxygen being produced. This is still somewhat debated.
The "Synthesis" Part
Basic. There are three phases.
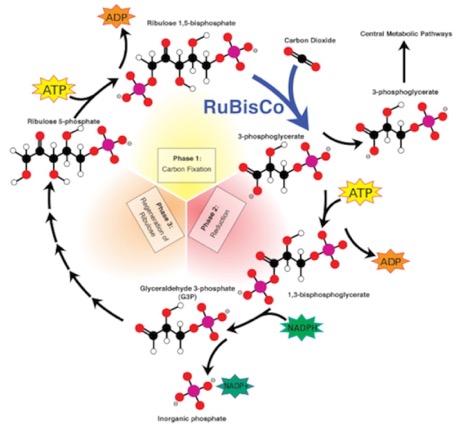
- Carbon Fixation: The starting molecule is Ribulose 1-5 bisphosphate, a 5-carbon sugar derivative with a phosphate on each end. CO2 is pulled from the air and attached to one end, creating a very unstable 6-carbon molecule that breaks almost immediately in to two 3-carbon molecules called 3 phosphogycerate. If you’re counting Carbons (and you should be), you started with 5, added 1 CO2 to get 6, then broke that into two 3-carbon molecules.
- Reduction: This molecule may be used for some biosynthetic pathways. However, to be really useful, it needs to be reduced from the carboxyl (COO-) form to the aldehyde form (C=O). This goes in two steps involving first ATP, then reduction with NADPH. This yields the very useful glyceraldehyde 3-phosphate (G3P). I’m sure you remember that one from glycolysis.
- Regeneration: For it to be a “cycle,” the starting material Ribulose, 1-5 bisphosphate has to be regenerated. This actually has several steps and if you don’t look a little at the details, the math doesn’t seem to work. For that reason, I’ll do a little detail here. What happens is the first two steps are run 3 times, (requiring 3 Ribulose 1-5 bisphosphate molecules), which gets you 6 G3P. One of those can be syphoned off to use for other things such as glucose synthesis. The remaining 5 G3P are rearranged through several enzymatic steps to get you back your 3 Ribulose 1-5 bisphosphate. (Five 3-carbon molecules get you three 5-carbon molecules…15 carbons in each case)
Detail
You don't need to memorize this. It's here for context. Please keep reading. There is more after this.
- We start with Ribulose 1-5 bisphosphate (that means 2 phosphates, one on each end of the molecule at positions 1 and 5). Note that ribulose is a ketose, with a carbonyl at position 2. The attack of the CO2 is at position 4, one from the right end.
- The intermediate, 3-keto-2-carboxyarabinitol-1,5-bisphosphate (hope you appreciate that I wrote out the name of the “β-keto intermediate”), is immediately broken into two 3-phosphoglycerates. These have a phosphate on position 3, a carboxyl group (acid) at position 1. Note that here they are assumed to be deprotonated, as they would be at normal pH.
- Phosphoglycerate can itself be used as starting material for many biosynthetic pathways. Some molecules may get syphoned off for other uses.
- ATP is used to add a phosphate to the other end (carbon 1) of 3-phosphoglycerate (3-PG), yielding 1,3 bisphosphoglycerate (you need two ATP because you have two 3-PG). Note that the carboxyl end (left side) now goes COOPO3-. In ATP hydrolysis, ADP would be left with the extra oxygen and the Pi picks up its fourth O from water. Here, the transferred Phosphate gains its fourth Oxygen from the carboxyl.
- NADPH is the reducing agent for the next step, which removes the phosphate. Note that if you now remove the Pi, the oxygen that was the end of the carboxyl is removed, leaving just the carbonyl. So, it’s now an aldehyde (terminal carbonyl) and is Glyceraldehyde 3-phosphate. It can be used in biosynthetic pathways too. The H from NADPH is now at the end of the molecule, where the O used to be.
- Once you build up enough G3P, you can syphon one off for Glucose synthesis (note that this particular version of the cycle implies that it’s the 3 phosphoglycerate that is syphoned off. This is a bit of a controversy...and may just be a point of view. Let’s stick with the G3P as being the main intermediate used to build glucose.
- I’m not going to go into any more detail on the regeneration process than I did above in phase 3.
CAM and C4 Plants
The problem of Oxygen
We discovered that Photosystem II has such an extremely high RedOx potential that it can oxidize Oxygen, removing it from water and releasing it as molecular O2. That’s good for us, but creates quite a few problems. The main problem as far as we are concerned here is that RuBisCO has a very odd property: it can carry out a very unproductive reaction called “photorespiration.” In this reaction, instead of binding CO2 and adding that to ribulose-bisphosphate (RuBP), it binds O2 and adds that to RuBP.
RuBisCO binds CO2 preferentially. But, if there is a lot of O2 around photorespiration happens.
The exact biochemistry is not that important here. There are a whole series of reactions that take place and the net result is that CO2 is released (that’s why it’s called “respiration;” oxygen is consumed and CO2 is released). But, unlike cellular respiration, ATP is consumed in the process. Thus, it is considered a wasteful process.
There is some debate how much of a problem this is for plants. Photorespiration may be helpful to some plants under some circumstances. However, it is clear that other plants have evolved specific strategies to minimize it.
Plants that have no strategy to prevent photorespiration are called C3 plants (because the product of carbon fixation are two, 3-carbon molecules…3 phosphoglycerate). They do photosynthesis the way I have taught it so far. They include most of our agriculturally important plants, such as wheat, rice, soybean, potato, fruit trees and nut trees and others. They tend to do well in cool, moist climates, but not at all well in hot, dry climates. In such climates, the plants will keep their stoma closed to conserve water. Gradually, this will deplete the CO2 and build up O2,) leading to photorespiration).
The Biochemistry
Plants that live in hot, dry climates have evolved mechanisms to prevent photorespiration. Neither evolution nor modern protein engineers have managed to produce a RuBisCo version that does not carry out photorespiration.
So, plants in hot, dry climates have taken a different approach: keep oxygen production away from RuBisCo and keep CO2 as high as possible around it.
One such type of plants is called C4 plants and the other CAM plants. Both these strategies involve keeping the concentration of CO2 relatively high when the Calvin Cycle is running. While there is some variation on these themes, the biochemistry is similar in both cases. I will present the simplest variant:
Both fix CO2 onto phosphoenolpyruvate (PEP) forming the 4-carbon molecule Oxaloacetate. That is converted to Malate by the enzymes indicated. You may remember from the Bass2 activity the references to PEP and C4 synthesis.
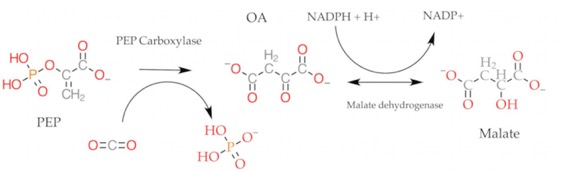
CO2 can be released from Malate, generating pyruvate. Remember “Pyruvate Kinase” from glycolysis? It will take pyruvate and add phosphate from ATP to regenerate PEP so the plant can do this again. So, in this case Pyruvate Kinase is doing what it's name says it does.
C4 Plants: Spatial separation.
These include a few important agricultural plants, such as corn and sugar cane as well as some grasses. “Crabgrass” is a C4 plant, which is why it takes over your lawn, especially in dry weather.
When grown in the same environment, at 30°C, C3 grasses lose approximately 833 molecules of water per CO2 molecule that is fixed, whereas C4 grasses lose only 277 water molecules per CO2 molecule fixed. This increased water use efficiency of C4 grasses means that soil moisture is conserved, allowing them to grow better in arid environments.
Thus, the grass we like requires roughly THREE TIMES the water as crabgrass. I still hate crabgrass.
C4 plants separate oxygen production from the Calvin cycle in two different sets of cells, known as the “mesophyll” and “bundle-sheath” cells, respectively. The set up looks like this:
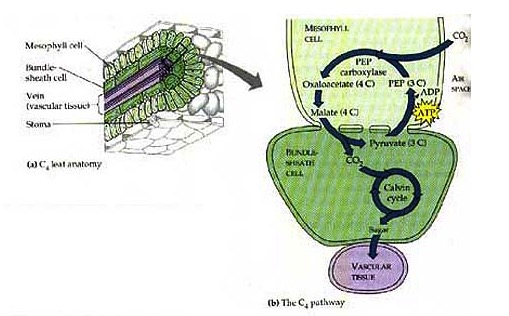
This image is used without permission from this website: http://www.bio.umass.edu/biology/conn.river/photosyn.html
They transport the malate made above into the bundle-sheath cells and released the CO2 in the chloroplast of those cells. This keeps the concentration of CO2 high in those cells and minimizes photorespiration. Another depiction (yes, I know it's in German) of the overall scheme is below:
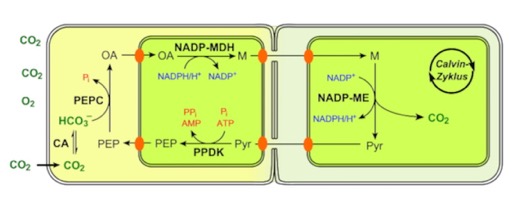
CAM Plants: Temporal separation
CAM stands for Crassulacean acid metabolism. There is no chemical named “Crassulacean acid.” The name comes from the family of plants that use this approach, Crassulaceae, and the fact that the chemicals oxaloacetate and malate are organic acids.
CAM plants include succulents. The only agriculturally important plants in this category are pineapple and agave.
They keep their stomata closed during the day, minimizing water loss. Then, at night, when temperatures are generally cooler, they open stomata and allow gas exchange. They save the Malate generated in the pathway above in the vacuole, then use it during the day to keep CO2 concentrations high. This image is from wikipedia and yes, the labels are again in German.
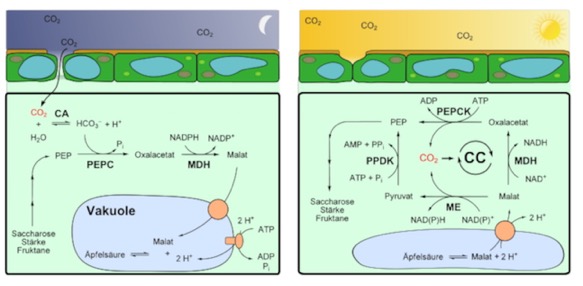
Side point:
PBS ran a documentary by Ken Burns not long ago on the “Dust Bowl,” in case any of you have trouble falling asleep, you can look it up. However, there is a good point to be made connecting this element of history to biology. The native grasses on the great southern plains, such as buffalo grass, Are C4 plants. These have typically deep roots and use less than ⅓ the water for each glucose generated than the shallow-rooted C3 plants, such as wheat, that we planted there in their place.